|
Most of what we know about
astrophysical magnetic fields has been detected via radio astronomical
observations. Most of the cosmic
broad-band (“continuum”) radio emission is synchrotron radiation by
relativistic electrons which spiral around magnetic field lines.
These electrons are probably accelerated in the remnants of supernova
explosions, together with other particles of the cosmic ray population.
The magnetic field originates from the dynamo
process driven by turbulent gas motions and/or cosmic rays. The
observed synchrotron luminosity allows us to measure the total
field strength. Synchrotron radiation is linearly polarised, up to 75%
in a fully regular magnetic field. The polarisation plane yields the
orientation of the regular field in the plane of the sky. The degree of
linear
polarisation tells us the field's degree of ordering. Moreover, Faraday
rotation of
the polarisation plane provides information on the field component
along the line of sight. Hence, a three-dimensional picture of cosmic
magnetic fields can be derived from radio waves.
|
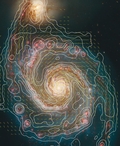
© A.
Fletcher & R. Beck (MPIfR)
|
This figure was
derived from combined
observations of the prototypical spiral galaxy M51 with the
interferometric radio telescope Very Large Array (VLA) near Socorro
(New Mexico/USA) and with the 100m single-dish radio telescope near
Effelsberg (Germany). The contour lines trace the total radio emission
at 4.8 GHz (6 cm wavelength) and the vectors the polarised emission. The
vectors indicate that the orientation of the regular magnetic field is
spiral and mostly follows the spiral arms, as evident from the
background optical image obtained by the Hubble Space Telescope. The
magnetic field is strong also between the spiral arms. This was not
expected from the idea that the spiral arms are density waves where gas
and magnetic fields should be compressed. Instead, the magnetic field
must be enhanced between the spiral arms by some other mechanism which
is still not understood. Outside of the optical extent of the galaxy,
little radio synchrotron
emission is detected at high frequencies because the relativistic
electrons, responsible for the radio emission and “illuminating” the
magnetic field, cannot
travel far away from the regions of their origin, supernova remnants.
These are embedded into the inner disk of galaxies where the star
formation rate is
highest. Only in a few cases, radio halos were detected around
galaxies seen edge-on.
|
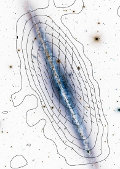
© M. Krause (MPIfR)
|
This
figure shows the spiral galaxy NGC
891,
seen almost edge-on, which
is believed to be very similar to our Milky Way. It was observed at 8.4
GHz (3.6
cm wavelength) with the
Effelsberg 100m telescope. The background optical image is from the
CFHT
Observatory. The "X-shaped" structure of the magnetic fields indicates
the action of a galactic wind. The observed extent of the radio halo is
limited by the large energy losses of the cosmic-ray electrons emitting
at this wavelength. At lower frequencies (longer wavelengths) the radio
waves are emitted by electrons with lower energies for which the energy
lossesare smaller, so that larger radio halos are expected.
|
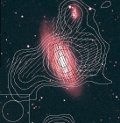
© C. Chyzy
(Jagiellonian Univ. Kraków)
|
In a
few spectacular cases the
radio synchrotron emission at high frequencies
is not restricted to the optical extent. This is always related to
interactions between galaxies. For example, huge radio lobes were
discovered on two sides of the galaxy NGC
4569 which is located in the
dense Virgo
cluster of galaxies where interactions are frequent. This figure shows
the
radio map observed at 4.8 GHz (6 cm wavelength) with the Effelsberg 100m
telescope. Many more cases of interaction tails and lobes are expected at lower frequencies. |